Springs are a critical component in most applications within our everyday lives and are often taken for granted as they are often hidden from view. Products that use springs in their operation, such as switches, valves, motion control, and many more, would have an erratic function and eventually fail without them.
Although they provide a vital function, most people rarely give a second thought as to how they are designed and made and the technology used in the manufacturing process. Read on to discover the essential aspects that our expert engineers consider when creating springs.
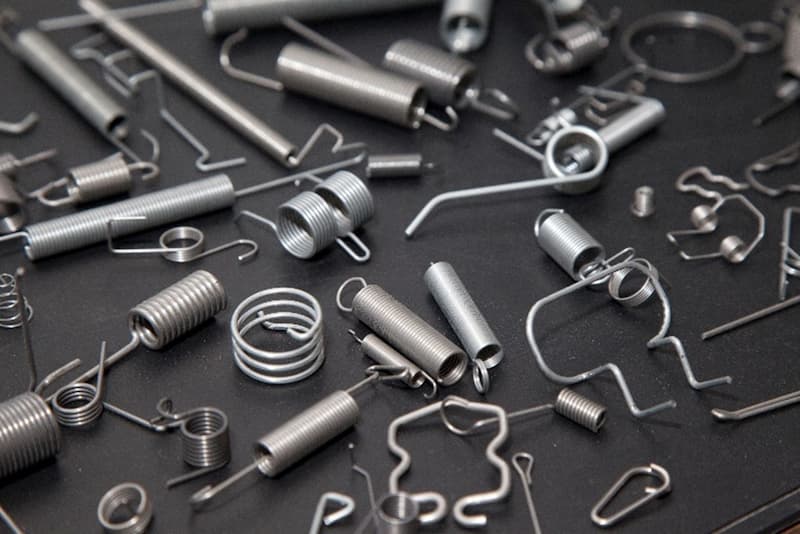
Anatomy of a Spring
Every spring, irrespective of type, stems from a basic anatomy that defines its functionality. Let’s explore the essential components that structure these tiny marvels of engineering: coils, ends, and dimensions.
Spring Coils
Coil count and coil size form the backbone of spring design. Coupled with material choice, they essentially dictate the spring’s capability. The coil count is the total number of coils present in a spring, which impacts the spring’s flexibility and strength. Higher coil counts generally equate to more flexibility.
The size or diameter of the coil also governs the spring’s characteristics. Smaller diameter springs offer more resistance to loads, proving useful in applications demanding strength over flexibility.
Spring End Types
Spring ends play a crucial role in how springs connect to the application. In compression springs, for instance, the ends are often designed to sit flat. There are several types of ends; here are a few examples:
- Closed squared ends are where the last coil (or two if it’s a double closed end) is bent parallel to the adjacent coil, making the spring end flat. Additionally, closed ends can be ground to make them perfectly flat.
- Open ends are not parallel and keep their helical form, but they can be ground as well to create a smooth end.
- Hooks and loops are sometimes chosen for designs like tension springs to allow the spring to be attached to the components that will generate the force it needs to combat.
Dimensions
The overall dimensions of a spring, including its length, total coils, and wire diameter, contribute significantly to its performance. Taking precise measurements ensures springs fit their applications perfectly, functioning with the efficacy intended.
Calculating Spring Rate
The spring rate, or stiffness, is another crucial factor in spring function. It is a measure of the force required to compress or extend a spring by a particular distance and is calculated using the formula:
Spring Rate (k) = Load (F) / Deflection (Δx)
And all together, that makes – F = kX
In practical terms, for a compression spring, if a force of 10N causes a deflection of 5mm, the spring rate is 10N/5mm = 2N/mm.
Understanding the anatomy of springs fosters greater success in selection and application. Through these fundamentals, at Airedale Springs, we drive precision in the intricate process of spring design, crafting meticulously to ensure our springs align with your needs.
The Elements in Spring Diameter Specifications
Because of the cylindrical nature of most spring designs, it is easy to assume the diameter relates to just the physical size of the spring in relation to the application it will be used for, but there is a lot more to it than that.
Outside Diameter (OD) and Inner Diameter (ID)
This relates mostly to the previously mentioned scale of the spring as a whole and will dictate how much space it will take up when compressed, at rest or extended. This data is found by measuring the outermost point of the coils, but the specifics will vary depending on the spring’s design. This data is the opposite; it is measured from the innermost points of the spring and relates to many spring applications that need to be installed over a rod or other external components.
Wire Diameter and Mean Diameter (d)
This relates to the diameter of the wire that makes the coil of the spring rather than the whole spring itself and is one of the most important parameters to address for spring design as it dictates how much force is needed to extend or compress the final spring.
Similar to the spring rate, this calculation is used to dictate the spring stiffness
The elements are the following:
- G is the absolute value (modulus) of rigidity of the spring material.
- d is the wire diameter (individually).
- D is the mean coil diameter (the middle of the inner and outer diameters measured from the centre).
- n is the number of active coils (not including closed coils like the ends connected to the application in question).
Using this formula, it is clear that the spring rate is directly proportional to the fourth power of the wire diameter. This means even small changes in the wire diameter can significantly affect the spring’s stiffness. These data points will influence many other areas of spring design, including load capacity, constraints and manufacturing considerations such as material use.
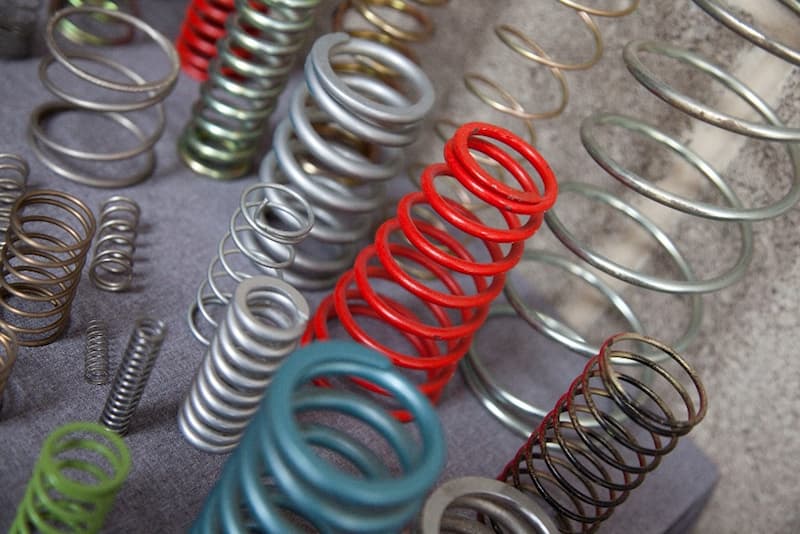
Spring Material Considerations for Reliable Design
Material selection is also a critical consideration. Different materials lend themselves to diverse applications; choosing the right one is paramount to enhancing durability and optimal functioning.
Stainless steel is often used as it is renowned for its corrosion resistance; this material is perfect for challenging environments that may involve exposure to elements or chemicals. Yet, it might not be suitable where high strength is needed due to its lower yield strength.
On the other hand, we have high-carbon steel. This material brings robustness and high fatigue resistance, which is ideal for automobile springs and other heavy-duty applications. However, its innate susceptibility to corrosion necessitates additional surface treatments for longevity.
Please note that options are not limited to these two. There are many alloys like Inconel or Hastelloy. These unsurpassed options offer exceptional resistance to extreme temperatures and corrosive environments. Yet, they come at a higher cost. This is why our engineers will often advocate their use when less expensive alternatives can’t settle application demands.
Defining Spring Stress and Fatigue Life
The stress experienced by the wire in a spring under load is also a function of the wire diameter. Thicker wires distribute the load over a larger cross-sectional area, reducing the stress on the material. This reduction in stress improves the fatigue life of the spring, making it more durable under repeated loading cycles.
The stress in springs can be estimated using formulas such as this
The elements of this are:
- τ\tauτ is the shear stress.
- F is the applied force.
- D is the mean coil diameter.
- d is the wire diameter.
Using this formula, we can see that stress is inversely proportional to the cube of the wire diameter. This means that increasing the wire diameter significantly reduces the stress for a given load. Generally, lower stress levels contribute to a longer fatigue life, reducing the risk of failure due to material fatigue. They are also effective for high-load applications and critical components used in aerospace and automotive spring design.
Bespoke Spring Design and Manufacturing at Airedale Springs
In the spring sector, “one size fits all” is a myth. At Airedale Springs, we embrace our role as a custom spring manufacturer because every application is unique, and we take great pride in offering a wide selection of spring designs.
We work closely with clients, ensuring our springs meet their specifications, whether that’s a small spring for a nifty electronic device or a robust compression spring for heavy machinery. The real essence of our work lies in developing our designs with your ideas to create the perfect spring solution for you. To learn more, contact us, and we’d be happy to assist you.